Understanding receiver specifications
Introduction
While the transmitter only generates the RF signal which is relatively straightforward to implement - receiving the signal and extracting the wanted information isn't. The ability of the receiver to isolate the wanted signal from unwanted signals is at the heart of its design and a poorly designed receiver can render a communication link unuseable.
By studying receiver specifications, the engineer can decide which parameters are important to increase the chances of creating a reliable communication link.
Important parameters for reliable communication
Receiver sensitivity
When radio waves strike the receiving antenna, a signal is produced at the receiver input. This signal has to be at a level that the receiver can work with in order for it to be demodulated. This minimum input level is the receiver sensitivity. If the signal input level becomes too low, communication can’t take place.
To measure sensitivity, data is modulated onto a carrier at a known frequency. This modulated carrier is connected directly to the receiver’s input. The receiver is tuned to the carrier and the input level adjusted.
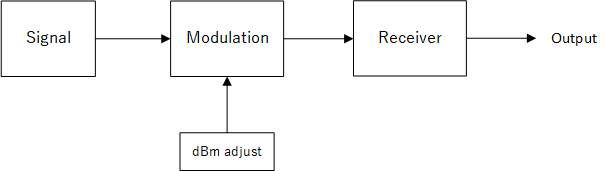
Measuring sensitivity
Sensitivity is expressed as the smallest power (dBm) or signal strength (dBuV) at the receiver input that is needed to produce a useable signal at the receiver output. The term "useable" can be qualified with an expression, such as "the input where the output bit error rate (BER) does not exceed 1%".
12 dB SINAD
For more details see this article, "What is 12 dB SINAD"
Another way of measuring sensitivity is determining the smallest level (commonly expressed in uV) that allows the receiver to output noise and distortion that does not exceed 25% (the 12 dB point) of the total signal. It is used in audio equipment for specifying readability of speech, but can be applied to any demodulated signal. In the case of radio modules, the "12 dB" is not relevant but an arbitrary value for comparison purposes.
Intermediate frequency
Let us consider the general topology of a radio receiver.
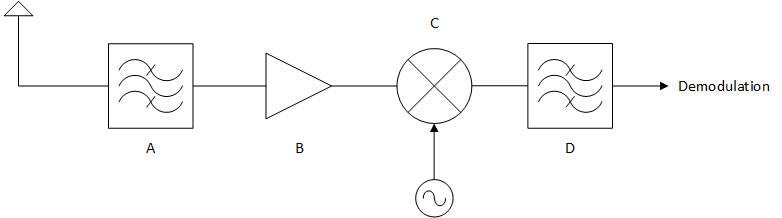
Receiver superheterodyne topology
The receiver needs to be sensitive enough and selective enough to be able to isolate the signal we want (which may also be at a very small level) which places greater and greater demands on the design of the receiver.
Receivers such as narrowband that are based around the superheterodyne configuration (see article here for more details on superheterodyne receivers) which in combination with a local oscillator, down converts our signal to an intermediate frequency or IF. The merits and problems of doing this are outlined in the linked article mentioned, but a receiver will be specified as having a first IF (and possibly a second IF / double superheterodyne).
Selection of IF
The values chosen to be used as the IF has become somewhat of a standard (e.g. 1st = 21.7 MHz, 2nd = 450 kHz), but generally it is a compromise between selectivity and the avoidance of image frequency. Why these are important, will be discussed further below.
Noise and interference
Noise is any undesired signal in our receiver that can interfere with reception of the main signal. They can be signals from other users (interference) or noise generated from the receiver's internal components (thermal noise). These cannot be prevented but suppressed as much as possible using filters.
As the radio spectrum becomes more congested, filtering is becoming increasingly significant. See a more detailed article on filters here.
Adjacent channel selectivity
The radio spectrum is divided into bands that are allocated to different applications (see here for an outline). The bands themselves are divided into defined frequency channels to create a band plan. The number of channels depends on the size of the band, the width of a channel (channel bandwidth) and how they are spaced (channel spacing).
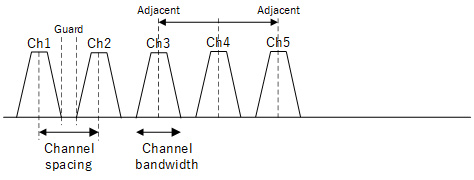
Band plan
Usually channels are spaced edge to edge meaning their spacing and bandwidth will always be identical, but in some cases a narrow space (or guard) can exist to act as a buffer. In this case the channel bandwidth and spacing will not be equal.
Now that we have a way of arranging communication into neat slots, there is no overlap and there should be no interference?
Recall that in a superheterodyne system, we use a bandpass filter ("D" in the topology diagram) to isolate the channel we are interested in receiving.
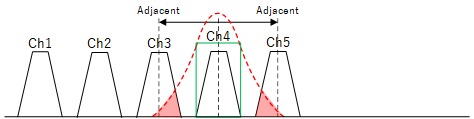
Adjacent channel selectivity
But real filters do have some roll off and allow some of the adjacent channel to "leak" into our wanted channel. Some receivers can suppress this interference more effectively and we measure this with a parameter called adjacent channel selectivity. We can measure this in 2 ways.
- The permitted ratio of the signal strength in the adjacent channel to the main signal (in dB). The higher the dB, the higher the adjacent signal selectivity.
- To obtain the permissible level (in dBm) of the signal in the adjacent channel, a defined signal level for the wanted signal in dBm must first be set. This level is specified in the corresponding technical standard (e.g. EN 300 220). The higher the dBm level of the signal in the adjacent channel, the higher the adjacent channel selectivity of the receiver.
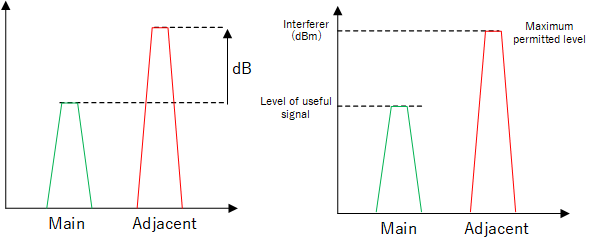
Measuring adjacent channel selectivity (relative and absolute)
Adjacent channel selectivity is critical if many channels are used in the same area. If you look at the graph on the right, it should be clear that a radio with higher sensitivity (lower dBm) can receive a distant signal - but without the corresponding increase in selectivity, adjacent channel transmitters would also have to reduce power or move further away to prevent interference.
This is known as the near far problem issue in radios.
Blocking performance
This is similar to what happens in adjacent channel selectivity, except now it measures the receiver's ability to suppress an interfering transmission happening in a nearby band. Since bands are individually regulated and do not have authority over other bands, such signal can be strong enough to overwhelm the receiver even if it's not on an adjacent channel. The ability of the receiver to suppress these out of band signals is called blocking performance. We can measure this in 2 ways:
- The permitted ratio of the out of band signal strength to the main signal (in dB) at a certain frequency offset in Hz. The higher the dB, the higher the blocking performance.
- The permitted strength of the out of band signal (in dBm) at a certain frequency offset in Hz. To measure the dBm value, there needs to be a level defined for the wanted signal beforehand. The higher the dBm, the higher the blocking performance.
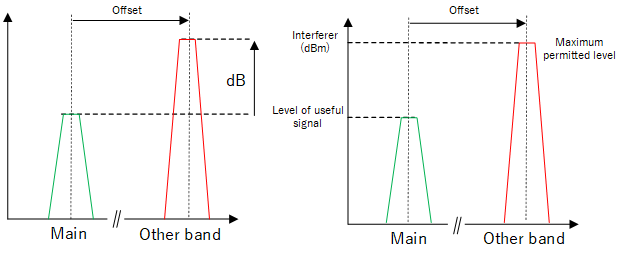
Measuring blocking (relative and absolute)
To improve blocking performance, we can place a filter ("A" in the topology diagram) at the first stage. The filter acts as a passband only for the channels the module is designed to receive. If the specifications emphasise this in-band filtering, you can be confident that any out of band signals will be sufficiently rejected.
Image frequency
If the out of band signal happens to be equal to the image frequency (see here for why image frequency exists) of the receiver, a second unwanted IF will of course be generated through the mixer and interfere with the main signal. The receiver's ability to suppress the image and other spurious signals while still being able to maintain communication comes under another category called spurious response rejection. Like blocking, it is expressed in dB or dBm.
Receiver key specifications summary
Parameter | Description |
---|---|
Sensitivity | This is the smallest input (in dBm) to the receiver while still being able to demodulate the signal. Increasing the separation between transmitter and receiver may reduce the input level below that is required for demodulation. |
Intermediate frequency | This is the frequency following downconversion from the primary RF frequency. If there are two downconversion stages, it is a "double superheterodyne" which allows for an increase in both channel selectivity and image rejection. |
Number of channels | Number of channels that can be selected in the receiver. A receiver may have channels across the whole band which allows a larger amount of transmitters to be used in an area. However the chances of interference is higher. |
Channel spacing | This is the distance from the current channel to the next receivable channel in kHz as described in the band plan. This is not always equal to the "channel step" which is the distance to the next receivable channel in the receiver's own channel table. |
Channel bandwidth | This is the width of a single channel in kHz |
Adjacent channel selectivity | This measures the receiver's ability to reject signals in the adjacent channels. |
Blocking performance | Blocking is the receiver's ability to suppress out of band signals. |
Channel table | The channel table contained in the receiver. If the channels are not selectable from a table, it will be from a set of discrete frequencies designed in by the manufacturer. |
Receiver classification
There is a method to classify receivers based on how well they fit into defined specifications. These specifications can be laid out officially in order that the manufacturers can qualify their receivers using a number system rather than having users sift through specifications.
The receiver category number system created by ETSI classifies receivers into category 1, 1.5 and 2.0 with "1" being the highest performing. See application note here for details.
Conclusion
It is tempting to decide a receiver based on the manufacturer’s specifications alone and then expect good performance.
However, specifications are only a method to compare one receiver to another based on measurements performed in laboratory conditions. This makes it a problem when trying to relate these figures to communication in the field as there are many variables (such as noise) in the environment which will affect the receiving capability.
A receiver may not perform equally in all areas. By understanding the kind of environment your receiver needs to operate, you can instead look for and focus on the most critical parameters in the specifications.